Not long after completing his Physics degree, Jason Fogg discovered the solution to a mystery in materials science. Soon he’ll be installing the hottest furnace in Australia for his research program at Curtin.
After high school, Dr Jason Fogg enrolled in a Bachelor of Science at Curtin, and now he’s a member of a world-renowned Curtin research team and a startup company, RapidGraphite, that is innovating the manufacture of a super substance – graphite. The most exciting part? Their work could be a game-changer for the lithium-ion battery industry.
What’s special about graphite?
Most of us first knew graphite as the stuff in the pencils we learned to write with. In fact, the word ‘graphite’ originates from the Greek language and translates as ‘to write’.
Graphite is a crystalline form of carbon. And while carbon isn’t a mineral, graphite is, and it’s found in metamorphic and igneous rocks all over the world. It’s common but unique – soft, greasy, chemically stable and able to withstand high temperatures. Crucially, it’s also an excellent conductor of electricity.
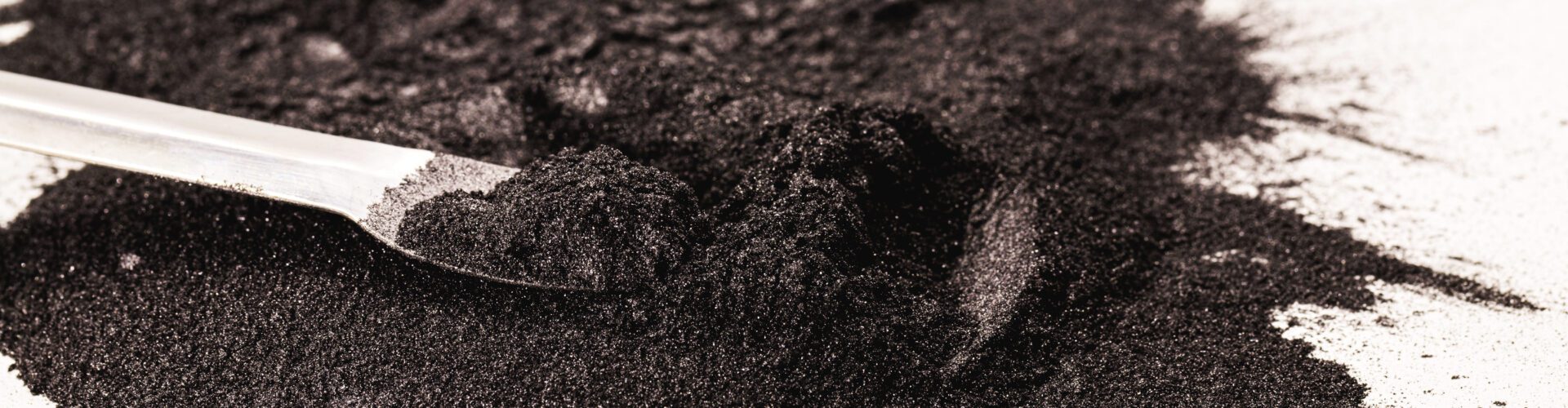
“Graphite’s atoms are layered and arranged in a hexagonal lattice, forming sheets. Each one of those sheets is called graphene,” Jason explains.
With all those advantages, graphite has abundant industrial uses beyond pencils – for lubricants, batteries, the production of glass and steel, the processing of iron, sports gear like tennis racquets, and much more. And because it’s chemically inert, graphite is even used in nuclear reactors to stabilise nuclear reactions.
But, as common as it is, graphite has been a bit of a mystery substance for material scientists for decades. Jason explains why:
“In the 1950s, a British scientist called Rosalind Franklin started researching graphite and how to make it. She proposed that all carbon materials when heated should form graphite quite easily. However, she showed that most carbon materials, when heated, actually don’t form graphite,” he says.
“And for the past 70 years it’s been a real question as to why they don’t.”
How to make graphite
Jason was working with graphite as part of his master degree research with the Curtin Carbon Group, intrigued with Rosalind Franklin’s physics puzzle, and continued to a PhD project.
“We were doing what we call blue-sky research,” he says. “We asked a fundamental question: why is it so difficult to make graphite?”
“We started with molecular dynamics. The Curtin Carbon Group has a lot of expertise in the simulation of graphite and how it forms.”
To explain that: Molecular dynamics are simulations of the behaviour of atoms and molecules, using high-speed computers – a technique that’s been likened to using a ‘virtual microscope’.
“Then we began to explore experimentally, which involved heating materials to extreme temperatures up to around 3,000 degrees Celsius.”
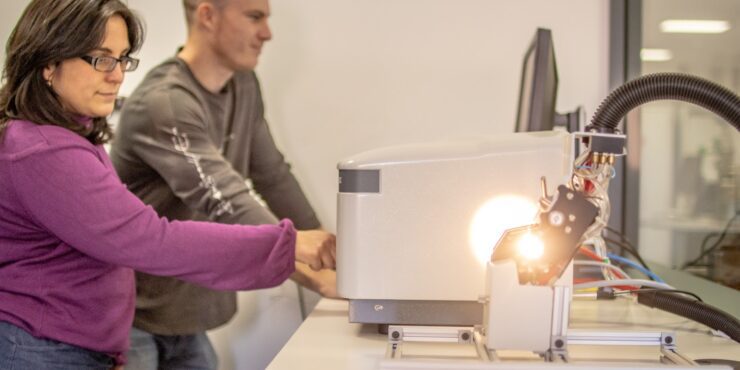
For perspective, 3,000 degrees is equal to about half the surface temperature of the Sun, and they were able to reach that temperature in seconds by ‘creating’ a customised furnace.
“We were on a shoestring budget – like most research starts out – so we repurposed an atomic absorption spectrometer. It’s an instrument that was invented in Australia in the 1950s and developed to analyse the composition of liquids.
“We sort of tweaked it so we could heat material to these wildly high temperatures that most furnaces can’t manage. Heating to 3,000 degrees so quickly allowed us to explore the kinetics of how graphite is formed.”
“We observed the first ever atomic-level detail of graphite forming, and watched that process essentially in snapshot frames over a couple of seconds.”
Does the world need more graphite?
Graphite isn’t especially difficult to mine or create, but both processes have industry challenges. For the manufacture of lithium-ion batteries, for example, graphite must be almost pure.
“You lose about 70–80% of what you dig out of the ground during purification, and the process uses a highly dangerous chemical – a single drop on your skin will kill you,” Jason says.
Or you can manufacture graphite. But the common manufacturing process for tonne-scale graphite hasn’t changed since the early 1900s and it’s extremely wasteful. It uses a lot of energy and about half is wasted, which contributes to significant CO2 emissions.
However, graphite is important in the manufacture of lithium-ion batteries, and their role in the green energy transition is driving a massive spike in demand for graphite. Complicating the increased demand, 98% of the world’s graphite supply for batteries comes from one country. This creates significant supply chain risk for other countries who need the mineral but can’t source it.
So, the best way to avoid supply disruptions is to create your own resource.
“The existing knowledge for manufacturing graphite starts with thermodynamics and a carbon phase diagram that shows that graphite should be easy to form.“
“But it’s only a select group of petrochemicals that can make synthetic graphite, so we started our research to better understand why thermodynamics doesn’t crossover into experimental results.”
The current theory on why most carbon materials don’t form graphite, is that internal defects prevent the material from restructuring into crystalline graphite even at temperatures as high as half the sun’s surface temperature.
The research team’s technology overcomes these defects, enabling them to produce graphite from materials, others would have considered as an impossible source for graphite.
“We’ve discovered specific catalysts blends that can take classic non-graphitising carbons and transform them into highly crystalline graphite – opening the door to new sources for this critical mineral.”
Interestingly, the innovative new method for making graphite is incredibly quick, taking just seconds to complete in the lab. Compared to traditional manufacturing methods that take days, this new method is a game changer for graphite production.
The logical next step was to establish a start-up company that could evolve the technology into an innovative business. The company RapidGraphite was registered and, through Curtin’s Accelerate program, the commercialisation journey began.
What will it mean for Australians?
One of the most exciting prospects is that the RapidGraphite process can quickly manufacture graphite for lithium-ion batteries, using a much more sustainable process.
“Graphite actually comprises about a quarter of the weight in all Li-ion batteries. So, a significant proportion of the make-up and the cost of every smart phone and smartwatch is graphite,” Jason explained.
But there’s a looming shortage for battery-grade graphite, predicted to reach about nine million tonnes by 2035, due to the rising demand for batteries needed to store energy from renewable sources. Could RapidGraphite solve that?
“RapidGraphite offers a revolutionary manufacturing process. Right now, we’ve got a simple aim of making low-cost graphite for Li-ion batteries. That would mean that batteries for home solar systems, for example, would be cheaper. “
“The graphite shortage also impacts on a national scale. Australia’s transition to net-zero will see large solar arrays built, and they’re going to need grid-scale battery storage. Most of the massive renewable resources that Australia has through wind and solar goes to waste – we have to store it, and we need graphite to make the batteries to store it.”
How does it feel to be a real-life innovator?
“It’s been a crazy ride. I was an undergrad student who continued on to research, and now we’re commercialising our research and taking into market,” Jason says.
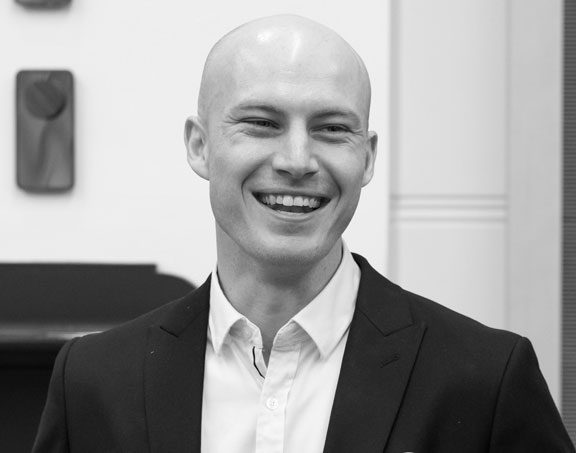
“Curtin’s Commercialisation team has been a massive support, and the Trailblazer funding program has just provided $500,000 for a new furnace. When it arrives around February 2025, it’s going to be the hottest furnace in an Australian university! It will allow us to scale our technology, take it out of the laboratory and into the commercial domain.
“It’s exciting, transitioning from that core research and carrying on with what I’m passionate about – which is making graphite.”