It’s long been accepted that all large objects in the solar system formed as a result of cosmic dust and water combining to make asteroids and comets, which were themselves the building blocks for planets. However, a numerical model that aligns with this widely accepted belief takes it in a slightly different direction – one that could make a significant impact.
Curtin University’s Professor Phil Bland has always found motivation in the fact that, unlike many other scientific fields such as chemistry and geology, the field of planetary science has no overarching theory that unifies existing theories.
“There are many components of planet formation, and as a community, we’re not at a place where there’s a general, unified theory that underpins how we go from basically dust and gas, which we can see with telescopes, all the way up to an orderly planetary system like we have now”, Bland explains.
“That story – and a lot of people don’t realise this – is really up for grabs.”
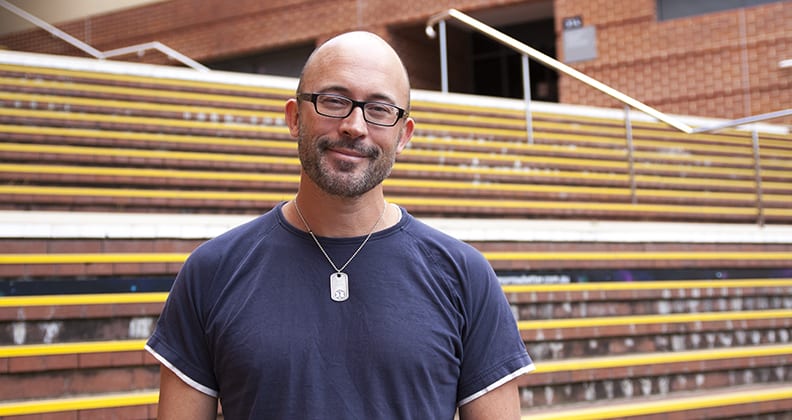
It was this quest for an all-encompassing theory that sparked an idea, a ‘thought experiment’ which led him to the conclusion that the asteroids that helped form larger planets actually began as mud, rather than rock, as previously thought.
“30 years of effort on the part of planetary scientists has not yet created a geophysical model that reconciles all the data on the geological evolution of ‘planetesimals’. I wondered if my ‘mudball’ idea might be the answer”, Bland says.
The Professor’s thought experiment revolves around the notion that, although we have rocky geological bodies now, they didn’t begin that way.
“Take the existing, widely accepted idea asteroids and comets (and some of the meteorites that come from them) began as ice and dust. Dirty snowballs of one sort or another – some asteroids just being a lot more dirt than snow. All we did was ask the question: ‘what happens to a dirty snowball when the snow melts?’”, Bland recalls.
“The first thing that happens in the evolution of that object is that it starts to warm up and melt the ice – at that point nothing has happened to turn it into a rock. It’s just a mixture of water and dust. And water and dust is mud. In this case, nice, warm mud, because of heat generated from short-lived radioisotopes that were kicking around in the early solar system. There’s convection going on inside that object, but it won’t look anything like what we would normally think of as a geological body. That’s the difference – we’re not considering rocky geological bodies. Other things happened before that”.
This thought experiment resulted in an unlikely collaboration with research colleagues on the opposite side of the theoretical viewpoint, and the globe. Dr Bryan Travis of the Planetary Science Institute, Arizona, and Prof Gerald Schubert of the University of California, Los Angeles (UCLA), had developed a numerical model which supported the existing view, and this posed a challenge for Bland when first establishing their research partnership.
“I had to write them an email that said ‘look guys, as you know, I think you’re wrong, but I’ve come to the conclusion that I’m also wrong. Here’s an idea for how maybe we could explain this.'”
“I had an intuition, but that’s not good enough. You have to know that the physics of it will work. And [Travis’ and Schuberts’] very beautiful, sophisticated, complex computer model could allow us to do that”, explains Bland.
“We’ve been on different sides of this argument in our papers, so I had to write them an email that said ‘look guys, as you know, I think you’re wrong, but I’ve come to the conclusion that I’m also wrong. Here’s an idea for how maybe we could explain this’”.
And so it began. Communicating predominantly via email and Skype, the researchers commenced testing the ‘mudball’ idea.
“We had a ‘first pass’ at the model in 2013. It didn’t include some pretty basic stuff, but it gave us enough of a feel that we were comfortable that we were on the right track. Over the next few years we added more, based on feedback from colleagues, mainly to get closer to approximate the reactions that go on when water reacts with unaltered rock to make clay”. That ‘first pass’ model, which showed that mud would convect, was a big deal because without convection the bodies don’t lose heat easily – they get too hot. After that it was iterative, adding more detail to more accurately get at the real physics of what was happening”, says Bland.
“What’s exciting is that it does seem to reconcile all the available data. I think we do have a model now for how this class of objects – the asteroids that contributed the water and organics to the terrestrial planets – formed and evolved”.
Bland continues to explore the theory in more detail with his research colleagues in the US, extending it to other meteorite groups in an effort to see if it’s a general geophysical model for the evolution of all small bodies in the solar system.
In addition to questioning the foundations of asteroids, the application of the model in a different context has helped identify differences in the composition and thermodynamic properties of asteroids and comets – insights that could have implications for space exploration beyond our solar system.
“I’m optimistic that we can extend this [theory] to make more informed predictions about what habitability might be like. We can apply components of this model to better understand the composition and geological history of an object that we see around another star”, reveals Bland.
“It’s very exciting for someone like me because it means that I might get to experience that wonderful moment, like geologists did from 1955 through to about 1970, where all of a sudden, before your eyes, everything clicks together. And that would be marvellous. And that’s why I got into it in the first place”.
Bland founded the Desert Fireball Network, which utilises a series of cameras placed around Australia to track the trajectory of meteorites, as well as the associated citizen science project, Fireballs in the Sky, which brings meteorite tracking to your smartphone. His plans for the future are equally as inspiring.
“One of my goals over the next five years is to have my planetary group within Curtin building instruments for NASA missions. I’d like to see us actually have a small mission. I think that’s absolutely achievable.”
Read the full research paper, ‘Giant convecting mud balls of the early solar system’.
To infinity and beyond: A space agency in Australia’s future
A Federal Government review of Australia’s defence and space capabilities is paving the way for an Australian space agency. Currently, we’re the only OECD country to not have one.
Having been a researcher within the field of planetary science for 25 years, and working within Australia for five, Professor Bland is excited at this development.
“An Australian space agency is the way to go. That will become the hub or office to connect people like me who do a combination of pure research and engineering with industry and defence”, he says.
He believes the shift towards creating an Australian space agency is significant because universities and industry will play an important role in designing and manufacturing the instruments and mission hardware fitted to spacecraft. But the role of these instruments doesn’t end there – they can then be repurposed.
“You’ve got technology transfer immediately from that mission that can go straight into industry”, Bland explains.
“By building a camera that goes on a Mars mission, and survives for 10 years in hard vacuum, using minimal power, NASA knows that the same camera can also go in 100 satellites”.
Bland also believes that the allocation of funding will be more efficient, and research collaboration easier than it is currently.
“Right now, it’s very hard to establish links between academia, industry, and defence – you spend a long time just trying to find the people to talk to. A space agency enables that, and allows government to strategically allocate funding to drive those collaborations. Everything clicks together pretty fast.”